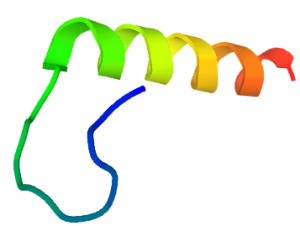
Short Peptide Folding
Short Peptide Folding
Understanding the process of peptide folding is a critical first step toward understanding protein folding. Depending on the temperature and solvent conditions, peptides are highly flexible and can adopt a variety of conformations in solution. Many unfolded peptides could spontaneously refold in vitro to form a native protein with full biological activity in the absence of other factors. Peptide fragments of proteins often have intrinsic propensities for the formation of their native conformations.
Proteins are the workhorses inside living cells. The interactions among proteins are critical for various important biological processes. Almost about 15-40% of the protein-protein interactions are peptide-mediated. A short stretch of amino acid residues from one protein partner contributes most to its binding to the other protein structure. These short linear interacting motifs can be found embedded inside disordered regions of intrinsically disordered proteins, or appear as flexible linkers connecting function regions and as flexible loops to rigid fragments and domains.
The primary sequence contains all the information to define the three-dimensional structure of a protein and its biological functions. The mutation or deletion of any amino acid may have a big impact on folding and stability. It takes nanoseconds (ns) for the peptide to form an intermolecular contact. The timescale of loop closing is 10 nanoseconds (ns). The formation of alpha-helical peptides is 200 ns, beta hairpins, and mini-proteins in 1–10 ms timescale. Many studies had a very good agreement between measured and calculated folding rates. Many factors such as temperature, pH, molecular chaperones, salts, and denaturants may affect a peptide in reaching its native state.
So it is critical to minimize factors that affect protein refolding. A successful folding should have inadequate denaturant concentrations to destabilize the native state of a peptide or protein. GuHCL can be used in order to disrupt the hydrophobic interactions within the tertiary structure.
References:
For refolding, proteins in the SDS-Polyacrylamide gels were incubated in transfer buffer I (0.01% Triton X-100, 48 mM Tris, 39 mM Glycine, 20%methanol, pH 9.2) twice for 15 min, and then transfer buffer II (48 mM Tris, 39 mM Glycine, 20%methanol, pH9.2) twice for 15 min, then transferred onto Immobilon-P membranes (Millipore) in transfer buffer II and processed by standard procedures for Western blots.
Reference: Zhou J, Blissard GW. Mapping the conformational epitope of a neutralizing antibody (AcV1) directed against the AcMNPV GP64 protein. Virology. 2006 Sep 1;352(2):427-37. doi: 10.1016/j.virol.2006.04.041. Epub 2006 Jun 14. PMID: 16777166; PMCID: PMC3767133.
Our Services:
Custom Peptide Synthesis Services
Other Posts: To Make Simpler and Better Biosensors LifeTein Peptides Used for Pulldown Assay Peptide Applications