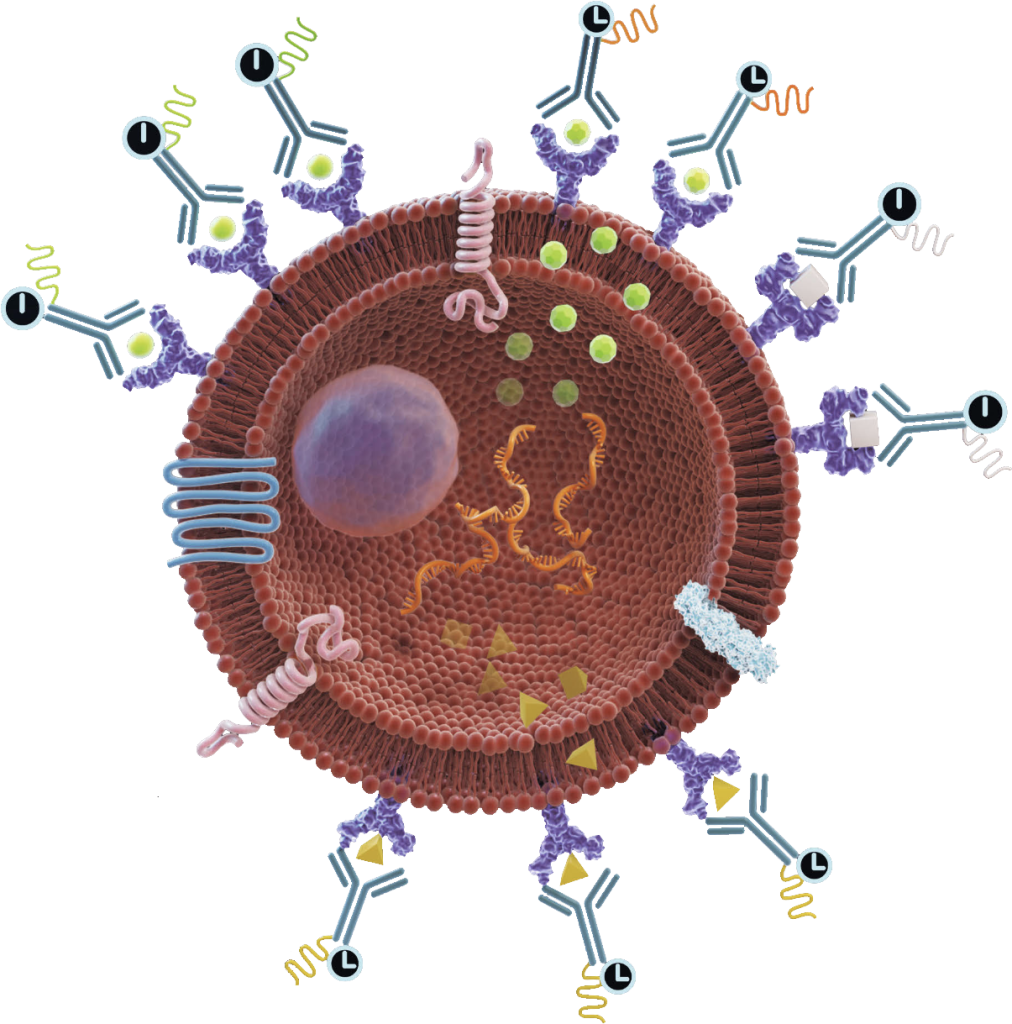
Live-cell imaging using peptides serves as an invaluable asset in the world of life science research, offering a window into the dynamic lives of cells in conditions closely resembling their natural habitat. By capturing real-time images of living cells, researchers can explore intricate aspects of cell behavior, growth, and movement.
In contrast to the static imaging of fixed cells and tissues, where photobleaching poses a significant challenge, live-cell imaging calls for a different approach. While fixed cell imaging demands high-intensity illumination and prolonged exposure, such conditions prove detrimental to the vitality of live cells. Consequently, live-cell microscopy finds itself in a constant balancing act, seeking to attain image quality while safeguarding cell well-being. This balance often imposes limitations on spatial and temporal resolutions in live-cell experiments.
Live-cell imaging encompasses a wide range of contrast-enhanced techniques in optical microscopy. The majority of investigations rely on various forms of fluorescence microscopy, often in conjunction with transmitted light methodologies. The ongoing evolution of imaging techniques and the development of fluorescent probes ensure that live-cell imaging retains its significance as a critical tool in the field of biology.
- The Fundamentals of Fluorescence Microscopy
One of the fundamental considerations is determining the precise amount of excitation light required to obtain a meaningful image. It’s worth noting that high-intensity light, especially in the near-UV range, can harm cells and potentially induce DNA damage. However, in live-cell fluorescence microscopy, the primary source of phototoxicity stems from the photobleaching of fluorophores. A critical protective measure involves deactivating the illuminating light when not in use. Employing shutters to control light exposure proves to be a pivotal element in live-cell imaging.
Additionally, it’s crucial to eliminate undesired wavelengths of light and opt for emission filters that are fine-tuned to maximize the signal. Mitigating photobleaching can be achieved by reducing oxygen levels, and minimizing background fluorescence can be accomplished by excluding phenol red and serum from the culture medium. It’s also essential to prevent any contamination of the illuminating light with even minute traces of ultraviolet or infrared wavelengths. The contained photobleaching chemistry within the β-barrel structure of fluorescent proteins (FPs) or peptides makes them less phototoxic.
The most effective strategy for reducing photobleaching and the associated photodamage is to minimize excitation light exposure by carefully managing exposure time and light intensity while maintaining a satisfactory signal-to-noise ratio tailored to the specific research question.
- The Live Cell Imaging Microscope
When selecting an optical microscopy system for live-cell imaging, three key factors come into play: detector sensitivity (signal-to-noise ratio), specimen viability, and the speed required for image acquisition. To optimize the signal-to-noise ratio, it’s crucial to select filters that closely match the spectral profiles of the fluorophores in use. Most epi-illumination microscopes and confocal systems acquire data in four dimensions.
Time-lapse imaging, involving the capture of cellular events over various timeframes, is commonly employed. This technique enables the repetitive imaging of cell cultures at specific time intervals. Wavelengths for excitation and emission filters should be finely tuned to match the fluorophore, thus reducing unnecessary light exposure. To minimize photodamage to the specimen, it’s advisable to use the lowest magnification that suits the specific experiment.
- Managing the Microscope Environment
Maintaining a physiological environment is imperative for cultured cells and tissues to behave naturally. Thus, the control of factors such as temperature and tissue culture medium composition plays a critical role in obtaining meaningful data in live-cell imaging experiments. Mammalian cell lines are typically maintained at 37°C. Variations in temperature and vibrations can negatively affect focus stability. To address these issues, options like stage-top incubators and fully enclosed microscopes can be considered. Most tissue culture media are buffered to a physiological pH using sodium bicarbonate and 5% CO2.
- Unmasking the Potential of Fluorescence Resonance Energy Transfer (FRET)
Fluorescence resonance energy transfer (FRET) is a remarkable technique that enhances the spatial resolution of fluorescence microscopes to under 10 nanometers. This substantial improvement in resolution makes FRET particularly appealing for studying co-localization events in biological samples, especially within living cells. However, in live-cell studies, the risk of FRET measurements being invalidated by acceptor fluorophore recovery, similar to FRAP experiments, must be carefully considered. Therefore, the use of acceptor photobleaching in live-cell experiments may not always be suitable.
Useful FRET calculator that provides a listing of key FRET pair information: www.fpbase.org/fret/
Furthermore, modern fluorescent dyes, such as the Alexa dye series, offer advantages like enhanced quantum efficiency and brightness, making them indispensable for specific techniques. LifeTein offers an extensive range of fluorescent labeling options, including FITC, FAM, TAMRA, Cyanine Dye Cy3, Cy3.5, Cy5, Cy5.5, Cy7, Cy7.5, EDANS/Dabcyl, MCA, AZDye, BODIPY FL or Alexa Fluor (Alexa488, Alexa532, Alexa546, Alexa594, Alexa633, Alexa647), ATTO Dyes (Atto465, Atto488, Atto495, Atto550, Atto647), and DyLight (DyLight 488, DyLight 550). However, chemical fluorescent dyes often exhibit higher cytotoxicity, possibly due to the cytoplasm’s reduced protection from reactive free radical breakdown products when compared to FPs, where the fluorophore is encapsulated within the FP beta-barrel structure. A range of cell-permeable fluorescent molecules is available for the specific labeling of intracellular organelles.
- Navigating Photobleaching
Photobleaching is an inherent aspect of live-cell imaging. Even the most advanced fluorescent molecules convert only a fraction of the absorbed energy into fluorescence. Some of this energy inevitably triggers chemical reactions that lead to fluorophore breakdown. Thus, aside from maintaining a physiological environment and confirming the specific labeling and function of the protein or organelle of interest, the most critical factor for successful live-cell imaging and obtaining meaningful data is the minimization of excitation light. This requires a thorough understanding of the microscope and optimization of components that regulate exposure, wavelength selection, and the collection of emitted photons.
A proper experimental setup is equally vital. Waiting for the visual system to adapt to darkness before attempting to locate a faint sample is advisable, given that modern cameras are often more sensitive than the human eye. However, it’s important to avoid using live camera modes to find your sample. Every photon is precious.
Unfortunately, photobleaching remains an unavoidable challenge, often determining the number of images that can be acquired. There is no simple solution to eliminate photobleaching completely. The specific excitation and emission bands, as well as the intracellular environment, influence the apparent brightness and, consequently, the apparent photobleaching of specific fluorescent proteins.”